The story of Jacutingaite: how a wonder material went from the mine to theory, crystal growth and experiments
by Nicola Nosengo, NCCR MARVEL
What does “success” mean for a project like NCCR MARVEL, that for 10 years has brought together experts from leading Swiss institutions to develop new computational tools for the study of novel materials? For sure, one key goal of the project has always been to prove that theory, computations and experiments can work hand in hand, resulting in something that is more than the sum of each part.
It was around that time that jacutingaite got on the radar of Enrico Giannini, an expert in quantum materials and crystal growth at the University of Geneva, where he leads the crystal growth facility. “In 2017 I was organizing the meeting of Swiss society for crystallography”, he recalls, “and I invited Nicola Marzari to introduce to our community how theorists were using crystallographic descriptions to create tools that could predict physical properties”. Marzari could not attend, but sent Marco Gibertini, at the time a member of his lab and now a professor in Modena and Reggio Emilia. “He gave a great talk, after which he told me that Antimo Marrazzo, at the time a PhD student in Marzari’s group at EPFL and now assistant professor at SISSA in Italy, had been going through the 2D database looking for topological materials, and he had found an incredibly exciting compound, but could not tell me much yet”.
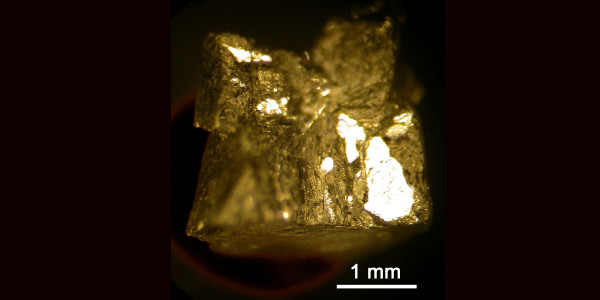
A crystal of Pt2HgSe3, or jacutingaite, grown in the Laboratory for Quantum Materials Discovery, DQMP, University of Geneva. Image from I. Cucchi et al., “Bulk and Surface Electronic Structure of the Dual-Topology Semimetal Pt2HgSe3” Phys.Rev.Lett 124, 106402 (2020).
Six months later, Marrazzo, Gibertini and Marzari were sure that they had calculated something reliable: monolayers of jacutingaite appeared to be a rare example of a quantum spin Hall insulator (QSHI), a class of so-called two-dimensional topological materials where the boundary acts as a conductor while the interior is an insulator —electrons, in other words, can only move along the edge of the material. In addition, electrons with opposite spin directions propagate towards opposite directions along the edge, an effect called spin-momentum locking. Even more remarkably, first-principle simulations showed that jacutingaite was the first ever material embodying the Kane-Mele physics with an energy scale such that could be potentially be observed at room temperature – but why was that so important?
This finding is related to the 2016 Nobel prize in Physics, that was assigned to Thouless, Haldane and Kosterlitz “for theoretical discoveries of topological phase transitions and topological phases of matter". In 1988, Haldane developed a theoretical model that was the first prototype of what we now call topological insulators: for the first time, topological matter was conceived to exist as an intrinsic property of materials, without external perturbations such as strong applied magnetic fields. Kane and Mele in 2005 showed that the magnetic field is not even necessary: graphene, which is made of a single honeycomb layer of carbon atoms, is a topological insulator, once relativistic effects are taken into account. However, relativistic effects are weak for light elements such as carbon, and the effect in graphene is way too small to be observed.
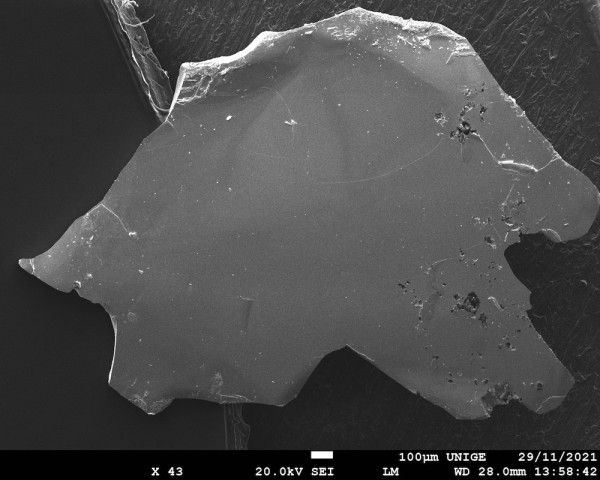
Switzerland-shaped crystal of jacutingaite grown in the Laboratory for Quantum Materials Discovery, DQMP, University of Geneva. Image from I. Cucchi et al., Phys.Rev.Lett 124, 106402 (2020).
Low-volume newsletters, targeted to the scientific and industrial communities.
Subscribe to our newsletter