Extending time-dependent density-functional theory to study magnetic excitations in transition-metal compounds
By Nicola Nosengo/NCCR MARVEL
Interesting phenomena happen when electrons in a crystal display collective behaviour. One example is a collective “spin excitation” that occurs when atoms in a solid are perturbed by an external magnetic field, which imparts a rotation to the atomic magnetic moments. These rotations can be represented as “magnetic waves” travelling through the crystal, which are quantized. A quantum of such collective spin excitations is called “magnon”. Magnons can appear in transition-metal and rare-earth oxides, and understanding and controlling them could potentially lead to technological applications in fields ranging from computation and memory storage to energy conversion. But despite numerous experimental studies of magnons in this class of materials, their theoretical description is still very challenging. A new article in npj Computational Materials by MARVEL members Luca Binci, Iurii Timrov and Nicola Marzari aims to fill this gap.
“As of now, there are not many methods available to calculate magnetic excitations in transition-metal oxides fully ab initio, without relying on simplified mathematical models, or without making arbitrary empirical assumptions” says first author Luca Binci, previously a member of the THEOS laboratory at EPFL and now at the University of California, Berkeley. One problem, he explains, is that density-functional theory (DFT) - the main tool for electronic-structure simulations of materials - is efficient at describing materials at their rest (i.e. in their “ground state” – the quantum state at the lowest energy level), but it becomes way more technically complicated whenever a form of excitation – including a magnetic one - is introduced. Another problem, Binci explains, comes from the very materials for which magnons are most relevant. “DFT works well for most of the periodic table, except for insulating compounds containing transition metals or rare earths. These materials form a large class where magnetic excitations are both technologically important and conceptually fascinating”.
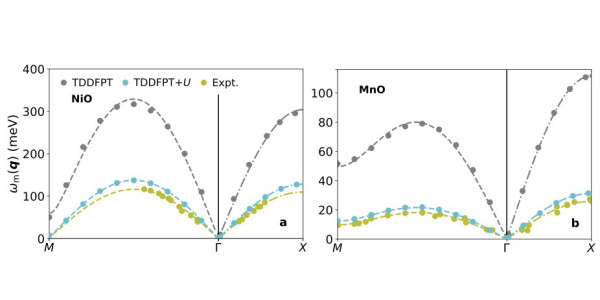
Calculated magnon dispersions with different methods and comparison with experiments. NiO and b MnO computed using TDDFPT (gray dots), TDDFPT+U (cyan dots), and as measured in experiments (olive dots) (Binci et al, npj Comput Mater, 2025).
To overcome these limitations, the authors of this study combined two extensions of DFT. The first one is DFT with Hubbard functionals (or DFT+U), that thanks to the introduction of an extra term in the calculation, the U correction, allows to properly account for the phenomenon of “electronic localization” occurring in insulating transition-metal or rare-earth compounds – a phenomenon that is generally poorly described by DFT with local and semilocal functionals. Over the last few years, the THEOS laboratory at EPFL headed by Prof. Nicola Marzari has pioneered techniques to calculate the U parameter from first principles reliably and efficiently, and these methodologies have been successfully applied to study these materials.
The second part is time-dependent DFT (TDDFT) - an extension of DFT that allows to describe the behaviour of materials in the presence of a source of excitation that varies in time.
“You can think of the ground state, or equilibrium state, as a sea with calm water” says Iurii Timrov, a scientist at the PSI Center for Scientific Computing, Theory, and Data at the Paul Scherrer Institut and last author of the paper. “But if you throw a stone in the water, you will see waves. In our case, instead of throwing a stone, we can irradiate the material with neutrons that scatter like billiard balls, and in doing so they lose energy and momentum within the material, and electrons are excited along their magnetic degrees of freedom. Because the kick from neutrons is time-dependent, our description of the resulting waves must also be time-dependent, and that’s why we use TDDFT.”
The new combination of DFT+U and TDDFT - termed TDDFT+U - was validated on manganese oxide and nickel oxide, two prototypical transition-metal oxides, and its predictions of magnon energies resulted in very good agreement with experimental results.
“We will use this method to help our experimental colleagues at the Paul Scherrer Institut to interpret their inelastic neutron scattering measurements on magnetic materials,” explains Timrov. Additionally, the team will study a class of magnetic materials called “altermagnets” that are currently receiving a lot of attention from scientists, and for which this method appears to be very promising. “Our current efforts in this direction are just the beginning, and our proposal to extend and apply TDDFT+U to altermagnets has been just accepted by the Swiss National Science Foundation, which has generously awarded us funding to hire two PhD students for this project and explore new frontiers in magnonics”, says Timrov.
For future studies, explain Binci and Timrov, the method could also be improved. On one side by making it faster by optimizing the code and extending the current implementation to GPU architectures, and on the other side by making also the U parameter time-dependent instead of fixed, which would allow to simulate ultrafast phenomena such as those occurring in pump-probe experiments.
Reference
Binci, L., Marzari, N. & Timrov, I. Magnons from time-dependent density-functional perturbation theory and nonempirical Hubbard functionals. npj Comput Mater 11, 100 (2025). https://doi.org/10.1038/s41524-025-01570-0
Low-volume newsletters, targeted to the scientific and industrial communities.
Subscribe to our newsletter